Sherman Zhao, MD, PhD
ABSTRACT
Currently it does not exist a full understanding of the underlying mechanisms of cancer. Two potential models to explain the development, maintenance, and recurrence of cancer include the stochastic model and the stem cell model. Recent insights into the function and characteristics of cancer stem cells (CSCs) offer a novel approach to understanding the generation, progression, and metastasis of cancer. A thorough understanding of the origins of cancer will generate new cancer research paradigms that can result in improved treatments. These treatments have the potential for better than current efficacy and less damage to patients than current.
Keywords
Cancer stem cells; Solid tumors; Therapeutic approaches
INTRODUCTION
Stem cells are immature, unspecialized cells that are capable of perpetuating themselves as stem cells and of undergoing differentiation into one or more specialized types of cells. Stem cells are most active during embryonic development and give rise to all the tissues in the body. Adult stem cells are found in differentiated tissues and can differentiate to form all the cell types in the tissue from which they originated. Stem cells are most commonly found in the bone marrow, where they give rise to the different blood cells. One of the most important functions of stem cells in postembryonic development is to repair and compensate for the loss of damaged tissues in the body. This dynamic process continues throughout life, and the unspecialized stem cells survive in each organ by creating their own niches. [1] Adult stem cells are thus central to tissue homeostasis and are identified through three distinctive properties: 1. Self-renewal: the ability to undergo division and form new cells with a potential identical to the mother cell. 2. Differentiation: the ability to give rise to a heterogeneous population of cells arranged in a hierarchical manner; includes various tissue-specific lineages, thereby building up the requisite critical mass toward replenishing the tissue of short-lived, differentiated cells. 3.Homeostasis: the ability to regulate and balance differentiation and self-renewal in the tissue or organ. [2, 3]
WHAT ARE CANCER STEM CELLS?
The first direct evidence for the existence of CSCs came from acute lymphocytic leukemia through extensive cell cloning and demonstration of their self-renewing capacity – a critical property of all stem cells. [4, 5] CSC identification in leukemia has, in fact, changed the way that many scientists view cancer and has led to their isolation from some solid tumors. CSCs represent only about 1% of the tumor but appear to be the only cells capable of generating a new tumor in immune compromised mouse models. [6] Whereas stem cells themselves maybe difficult to isolate from an already differentiated healthy tissue such as the breast, it has been possible to isolate stem cell–derived clonal tumor cells by engrafting tumor tissues in suitable animal models such as breast tumor–derived cells transplanted in the mammary fat pads of non obese diabetic/severely compromised immunodeficient mice (NOD/SCID).[7]
The existence of cancer stem cells had been hypothesized for many decades but was not until 1997 that they were isolated from patients with acute myeloid leukemia. Subsequently, cancer stem cells have been isolated from breast and brain cancers, and cancer cell lines. [8, 9] Cancer stem cells may derive from mutations in normal stem cells. Alternatively, differentiated tumor cells may acquire the characteristics of stem cells. Cancer stem cells are present in only very small numbers in tumors and may not be present in all tumors. [7, 10, 11]
THE SIGNIFICANCE OF CANCER STEM CELLS
Current therapies treat tumors as if all cancer cells had the ability to proliferate and metastasize. Typically, the therapy shrinks the tumor and appears to be successful, but then the tumor grows back. The therapy killed the susceptible cells, but the resistant stem cells remained.
Stem cells have many of the characteristics of cancer cells. They share the ability for self-renewing cell division, and resistance to apoptosis (programmed cell death). Several of the molecular signaling pathways associated with normal stem cell development, such as Wnt, Shh and Notch, are also active in cancer development. Stem cells are notable for the presence of ATP-binding cassette transporters, which remove drugs from the cell.[3] Cancer stem cells maintain this characteristic, which accounts for the ineffectiveness of chemotherapy to destroy these cells. Normal stem cells are under tight metabolic control and divide only under specific conditions. Cancer stem cells no longer have these controls. [12-14]
Possible origins of CSCs in tissues leading to the occurrence of cancer which include: Transformation of stem cells residing in a tissue, leading to altered growth and differentiation properties; Transformation of a local pool of early progenitors that re-acquire self-renewal properties; Series of effective mutations that render committed transient-amplifying progenitor or differentiated somatic cells within a tissue immortal (de-differentiation); Fusion of circulating bone marrow–derived stem cells with tissue-residing cells.[15]
Characteristics shared by normal and cancer stem cells are: Capacity for asymmetric divisions (self-renewal), which generates a quiescent stem cell and a committed progenitor and contributes toward developing a critical mass of cells. Regulation of self-renewal by similar signalling pathways (Wnt, Sonic Hedgehog, and Notch) and at the epigenetic level by Polycomb genes (BMI-1andEZH2). Expression of factors such as Oct4, Nanog, and Sox2 and also nodal and cancer test is specific antigens (CTAs), which maintain a functional plasticity by promoting pluripotency and immortality. Capacity to arrange a hierarchy of cellular derivatives that includes progenitors and differentiating cells. Extended telomeres and telomerase activity that increases the cellular lifespan. Expression of ABC transporters, contributing to cellular resistance against specific growth-inhibitory drugs. Predisposition for growth factor independence through secretion of growth factors and cytokines. Stimulation of angiogenesis through secretion of angiopoietin factors. Expression of similar surface receptors (e.g., CXCR4, CD133, 6 integrin, c-kit, c-met, LIF-R) that are either identified as stem cell markers or are associated with homing and metastases.[16]
It is also to be expected that the role of CSCs in migration and metastases would be much different than their role in primary tumors. Such differential regulation of migrating CSCs and those in metastases is also not yet identified.[17] Once additional insights into the biology of candidate CSCs, as well as of those subpopulations that initiate metastases, become apparent with further characterization and validation of their gene and protein expression profiles, as well as the role of these molecules in tumor progression and clinical responses, it will become clear whether engrafting activity (the key feature of identification protocols today) is an accurate reflection of stem cell activity.[18] However, it is certain that advances in our knowledge of the properties of stem cells have definitely culminated in a realization for specific targeting and eradication of CSCs, and that treatment of bulk disease is an insufficient panacea for cancer. [19-22] The development of a new generation of treatments to target the rare CSCs is thus critical, but poses formidable challenges: Ideally, a therapy should target unique CSC pathways and “turn back the clock” from a state of disease to one of normal tissue and organ homeostasis.
A concern in achieving the goal stated above is that normal stem and progenitor cells actually prove to be more sensitive than CSCs to the effects of current chemotherapeutic drugs. This provides a competitive advantage to CSCs and makes their positive selection quite likely, leading to the emergence of drug resistant clones. Delineation of the effects of the new drug regimes on the evolution of CSCs is thereby imperative.
In cases where clinical remission is achieved, the presence of drug-resistant CSCs that have “escaped” chemotherapy would initiate a relapse. This necessitates the development of sensitive methods for detection of residual CSCs for follow-up in patients in remission. The establishment of diagnostic endpoints by which treatment success can be measured is thus required. A culmination of the understanding of CSC biology will thus aid the development of more effective and targeted therapies to treat this astoundingly complex and devastating disease. [22, 23]
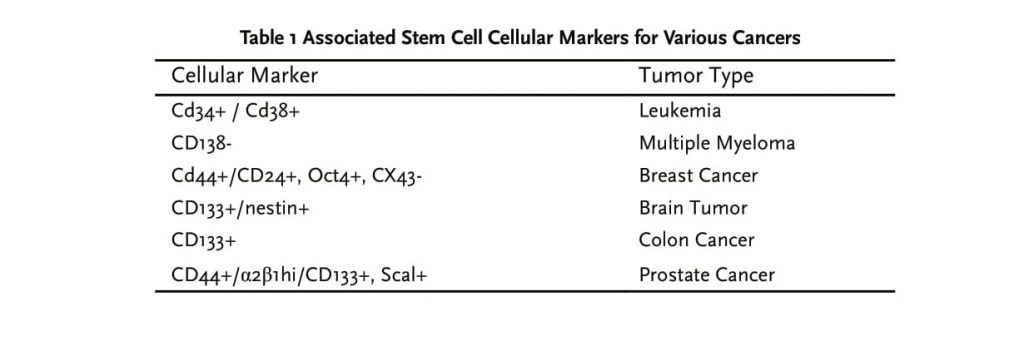
CANCER STEM CELLS AND THE METASTATIC
This fact gives the idea that a single cancer cell can drive the formation of a metastatic tumor, [24] CSCs are likely responsible for distant tumorigenesis as they are in primary tumor formation. The incongruity between the large number of malignant cells found in the vascular systems of cancer patients and the comparatively small number of macro-metastases can be reconciled by the existence of mCSCs. Though other cell subpopulations may break free of the primary tumor and invade the blood stream, mCSCs, like their pCSC counterparts, are those solely responsible for initiation of tumors. mCSCs are related to pCSCs in essential properties of self-renewal and differentiation needed for the propagation of the bulk of the tumor but differ in keyways. Unlike pCSCs, mCSCs disseminate from the tumor, colonize foreign tissue, and likely have additional alterations (whether mutational, epigenetic, or adaptive) which allow survival and propagation in secondary sites. [25-27] This has been shown in pancreatic cancer where mCSCs express the same stem cell marker CD133+ as pCSCs, but additionally express a novel CXCR4 marker known to specifically effect migration and homing of metastatic cells. The fact that CSCs can be isolated, not only from the primary tumor, but also from pleural effusions and metastases indicates that these cells have the capacity to migrate and invade other tissue. A common early indicator of metastatic breast cancer is the presence of breast cancer cells in the bones, which in more than 50% of cancers display the CD44+/CD24 expression profile associated with CSCs. This strongly suggests existence of mCSCs capable of proliferating in the bone marrow, the most common site of breast cancer metastasis. It should be noted, however, that pioneering studies to identify pCSC markers in breast cancer assayed cells from pleural effusions, not primary tumors. Recent research has focused on endpoints of metastasis measured by expansion of the secondary tumor, other steps of the metastatic cascade must affect mCSCs as well. Processes of Angiogenesis, invasion into surrounding stroma, intravasation into lymphatics or blood vessels, adhesion to luminal epithelia, and extravasation should all be explored with regards to mCSC function. This would provide insight into mCSC function and tissue tropisms as well as the process of metastasis in general. Understanding tumor dissemination at a mechanistic level, especially with regards to mCSCs will reveal new potential intervention points for treatment as well as new criteria for diagnosis.
TREATMENT AND PREVENTION
Surface markers may not always be the optimal strategy for eliminating malignant stem cells. Some research has begun to focus on mechanistic differences between normal and malignant stem cells. Similar to the research done to understand how cancer develops, the first work to identify mechanistic differences examined leukemia. Their intriguing finding was that normal hematopoietic stem cells depend on the PTEN suppressor gene, while leukemia stem cells in mouse models do not. Conveniently, there is also a treatment, rapamycin, with potential benefit in this situation. In leukemia, the cells produce mTOR to mediate the effects of the deleted PTEN gene. Rapamycin inhibits mTOR. In experiments that treated mice with rapamycin, the result was the elimination of leukemia and restoration of normal hematopoiesis. [28] This is only one mechanistic difference between normal and malignant stem cells, but it provides hope that further research will reveal other therapeutic differences.[29] Another potential avenue for therapeutic targets is the activated signalling pathways, such as Notch and Hedgehog. These signalling pathways are associated with certain malignant stem cells. In the Notch pathway, the processing of the enzyme γ-secretase regulates many of its effects. Inhibition of this enzyme by GSI led to a decreased number of proliferating transformed human fibroblasts in both in vitro and in vivo studies. Another potential avenue for decreasing Notch signalling pathway activity is to activate the antagonistic Numb signalling pathway. Activation of the Hedgehog signalling pathway provides another avenue for therapy. Constitutive expression of Hedgehog in the prostate is associated with the development of highly metastatic malignancy. A concern in this situation is that healthy prostate epithelium still expresses Hedgehog at regulated levels. As a result, complete inhibition of Hedgehog is not a valid therapeutic option.[30] A potential target that differs between malignant and benign cells is the expression of Smoothened, a responsive element. Currently, there are no concrete methods for monitoring or manipulating the Hedgehog pathway for treating prostate cancer. One promising study showed that cyclopamine, a Hedgehog inhibitor, led to complete regression of human prostate tumors in athymic mice. In transgenic mouse models for medulloblastoma the molecular inhibitor HhAntag bound to Smoothened with higher affinity than cyclopamine and thus could inhibit signalling in neuroblastoma cells. [31, 32] The current state of knowledge for treating the effects of stem cells in cancer is expanding. Current research shows that stem cells have a specific role in forming tumors. Researchers are using these pathways to develop very specific and effective targets for cancer therapy. Recognizing that stem cells play a central role in the development, maintenance, and metastasis of cancer provided a wealth of knowledge, which will hopefully lead to a promising treatment that combats cancer. [27, 33, 34]
FUTURE PERSPECTIVES
The identification of cancer stem cells in solid tumors has important implications for basic cancer research. Most analyses of tumors, such as gene expression, microarrays, proteomic, and many phenotypic assays, have been performed on whole tumors and have not revealed data on the small fraction of tumor stem cells. In addition, screens for cancer cytotoxic drugs have involved cell cultures treated over short time periods. Drugs specifically targeting cancer stem cells may display modest activity in short-term proliferation assays and be rejected for further follow-up study in animals or humans. Several important questions remain from the current data. Do the side population cells isolated from cell lines bear a relationship to cancer stem cells? In principle, in any permanent cell line there must be a self-renewing cell population. If the characterization of the SP cell sin cell lines could be applied to cancer stem cells, this could advance understanding rapidly. One property of cancer cells, like stem cells, is their ability to grow in soft agar cultures. It has been found that only a fraction of cells in a tumor cell culture can form a colony in soft agar. Are the cells forming soft agar colonies cancer stem cells? This would be a logical conclusion from the information at hand. It is known that the clonogenicity varies substantially between different tumor cell lines. If clonogenicity it related to self-renewing cells in the culture, assays based on colony formation maybe useful for screening for stem cell targeting therapies. Such assays would be more time consuming and have a lower throughput but might in the end prove more informative. Metastasis is the most troublesome property of tumor cells. The vast majority of cancer fatalities are due to the effect of the spread of the initial tumor to other sites. Although they can be quite invasive, basal cell carcinomas virtually never metastasize and are rarely fatal. Stem cells, especially certain cells of the neural crest, possess the ability to migrate through the developing embryo. The neural crest gives rise to the precursors of melanoma, neuroblastoma, and small cell lung cancers, all highly metastatic tumors. Is the metastatic ability of tumor cells related to an innate property of the cancer stem cells to migrate? If true, further characterization of germ cell migration could lead to new insights into metastasis. Anti–cancer stem cell therapies might find their best application in the restriction of metastasis. If metastasis could be prevented, even if the primary tumor remains intact, the patient might still experience a substantial increase in survival time.
CONCLUSION
Over the last several years, the cancer stem cell theory has gone from mere speculation to a highly evidenced working model of tumorigenesis. Regardless of whether these CSCs come from adult stem cells or progenitors, it is clear that they have a role in initial tumor formation and in metastasis. Understanding CSCs on a molecular, tissue, and organism level is crucial to understanding the true nature of cancer. Starting with better diagnostics based on CSC markers and eventually targeting drugs top CSCs and mCSCs will undoubtedly result in improved prevention and treatment of many types of cancer.
REFERENCES
1. Hombach-Klonisch, S., et al. Cancer stem cells as targets for cancer therapy: selected cancers as examples. Arch Immunol Ther Exp (Warsz), 2008; 56(3): 165-80.
2. Bosch Barrera, J., et al. Stem cells and cancer: elucidating the origin of the cancer stem cell. Rev Med Univ Navarra, 2007; 51(2): 14-7.
3. Li, L. and Neaves, W.B. Normal stem cells and cancer stem cells: the niche matters. Cancer Res, 2006; 66(9): 4553-7.
4. Anichini, A. and Cascinelli, N. Stem cells research: impact on molecular biology of cancer and prospects for therapy of neoplastic diseases. Tumori, 2000; 86(5): 375-80.
5. Huntly, B.J. and Gilliland, D.G. Leukaemia stem cells and the evolution of cancer-stem-cell research. Nat Rev Cancer, 2005; 5(4): 311-21.
6. Manzo, G. Cancer genesis: stem tumour cells as an MHC-null/HSP70 – very high ‘primordial self’ escaping both MHC-restricted and MHC-non- restricted immune surveillance. Med Hypotheses, 2001; 56(6): 724-30.
7. Trosko, J.E., et al. The role of human adult stem cells and cell-cell communication in cancer chemoprevention and chemotherapy strategies. Mutat Res, 2005; 591(1-2): 187-97.
8. Collins, A.T. and Maitland, N.J. Prostate cancer stem cells. Eur J Cancer, 2006; 42(9): 1213-8.
9. Lynch, M.D., Cariati, M. and Purushotham, A.D. Breast cancer, stem cells and prospects for therapy. Breast Cancer Res, 2006; 8(3): 211.
10. Huntly, B.J. and Gilliland, D.G. Cancer biology: summing up cancer stem cells. Nature, 2005; 435(7046): 1169-70.
11. Koeneman, K.S., Prostate cancer stem cells, telomerase biology, epigenetic modifiers, and molecular systemic therapy for the androgen-independent lethal phenotype. Urol Oncol, 2006; 24(2): 119-21.
12. Polyak, K. and Hahn, W.C. Roots and stems: stem cells in cancer. Nat Med, 2006; 12(3): 296-300.
13. Tataria, M., Perryman, S.V. and Sylvester, K.G. Stem cells: tissue regeneration and cancer. Semin Pediatr Surg, 2006; 15(4): 284-92.
14. Wicha, M.S., Liu, S. and Dontu, G. Cancer stem cells: an old idea–a paradigm shift. Cancer Res, 2006; 66(4): 1883-90; discussion 1895-6.
15. Dalerba, P., Cho, R.W. and Clarke, M.F. Cancer stem cells: models and concepts. Annu Rev Med, 2007; 58: 267-84.
16. Sales, K.M., Winslet, M.C. and Seifalian, A.M. Stem cells and cancer: an overview. Stem Cell Rev, 2007; 3(4): 249-55.
17. Burleigh, A.R., Of germ cells, trophoblasts, and cancer stem cells. Integr Cancer Ther, 2008; 7(4): 276-81.
18. Fodde, R., Stem cells and metastatic cancer: fatal attraction? PLoS Med, 2006; 3(12): e482.
19. Al-Hajj, M., Cancer stem cells and oncology therapeutics. Curr Opin Oncol, 2007; 19(1): 61-4.
20. Sehl, M.E., et al., Differential destruction of stem cells: implications for targeted cancer stem cell therapy. Cancer Res, 2009; 69(24): 9481-9.
21. Thenappan, A., et al., New Therapeutics Targeting Colon Cancer Stem Cells. Curr Colorectal Cancer Rep, 2009; 5(4): 209.
22. Winquist, R.J., et al., Targeting cancer stem cells for more effective therapies: Taking out cancer’s locomotive engine. Biochem Pharmacol, 2009; 78(4): 326-34.
23. Tu, L.C., et al., Targeting stem cells-clinical implications for cancer therapy. Curr Stem Cell Res Ther, 2009; 4(2): 147-53.
24. Jasmin, C., [Tumor stem cells and implications for the immunotherapy of cancer]. Comp Immunol Microbiol Infect Dis, 1986; 9(2-3): 253-61.
25. Yi, S.Y. and Nan, K.J. Tumor-initiating stem cells in liver cancer. Cancer Biol Ther, 2008; 7(3): 325-30.
26. Besancon, R., et al., Cancer stem cells: the emerging challenge of drug targeting. Curr Med Chem, 2009; 16(4): 394-416.
27. Jones, R.J., Cancer stem cells-clinical relevance. J Mol Med, 2009; 87(11): 1105-10.
28. Passegue, E., Rafii, S. and Herlyn, M. Cancer stem cells are everywhere. Nat Med, 2009; 15(1): 23.
29. Sasportas, L.S., et al., Assessment of therapeutic efficacy and fate of engineered human mesenchymal stem cells for cancer therapy. Proc Natl Acad Sci U S A, 2009; 106(12): 4822-7.
30. Short, J.J. and Curiel, D.T. Oncolytic adenoviruses targeted to cancer stem cells. Mol Cancer Ther, 2009; 8(8): 2096-102.
31. Wakimoto, H., et al., Human glioblastoma-derived cancer stem cells: establishment of invasive glioma models and treatment with oncolytic herpes simplex virus vectors. Cancer Res, 2009; 69(8): 3472-81.
32. Piccirillo, S.G., et al., Brain cancer stem cells. J Mol Med, 2009; 87(11): 1087-95.
33. Marotta, L.L. and Polyak, K. Cancer stem cells: a model in the making. Curr Opin Genet Dev, 2009; 19(1): 44-50.
34. Iwasaki, H. and Suda, T. Cancer stem cells and their niche. Cancer Sci, 2009; 100 (7):1166-72.